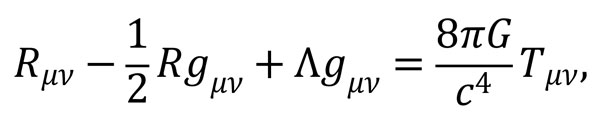
Physicists and Astronomers often say that matter tells spacetime how to curve, and curved spacetime tells matter how to move.
Figure 1 illustrates how the distribution of energy/matter will curve spacetime. The more massive the object, the more it will curve spacetime.
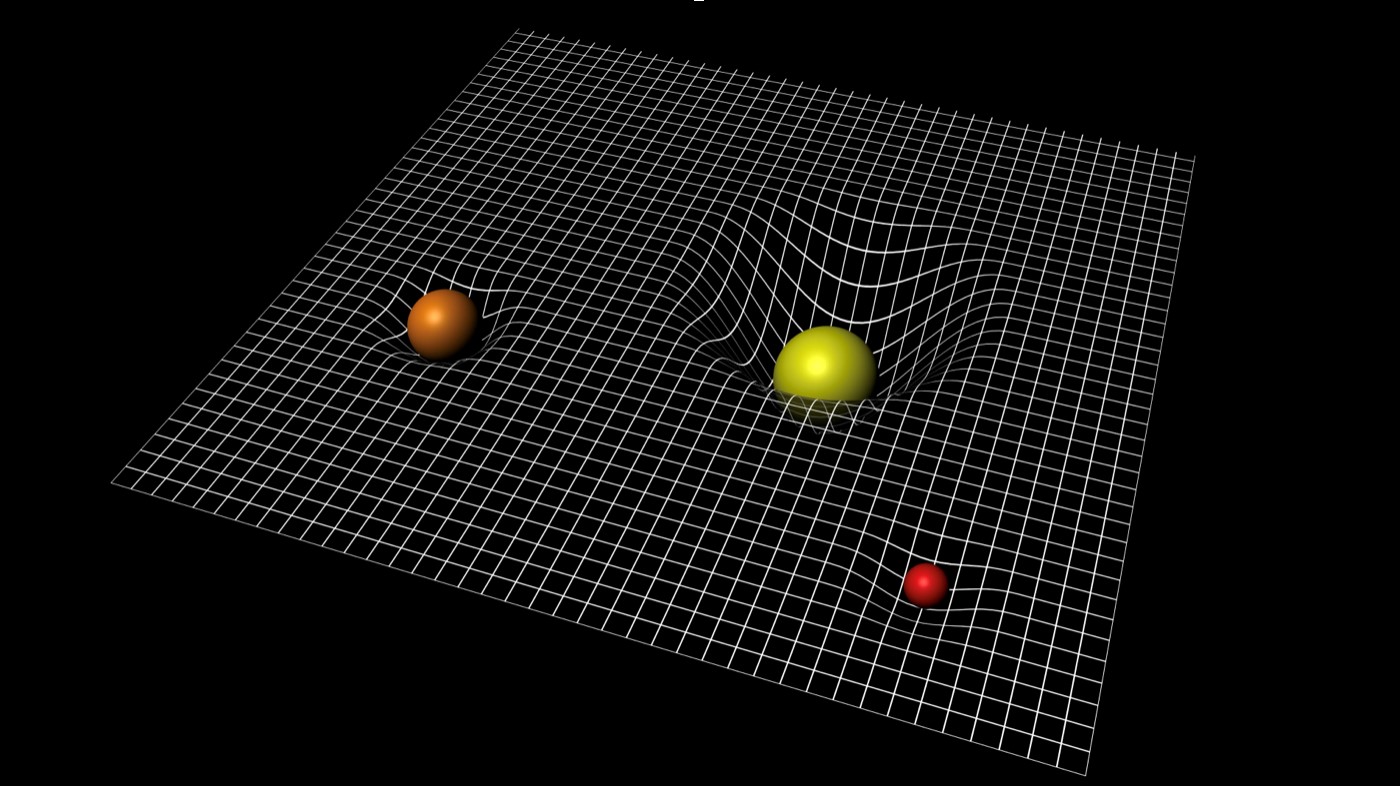
Figure 1: How different masses curve the fabric of spacetime. Image credit: ESA-C.Carreau
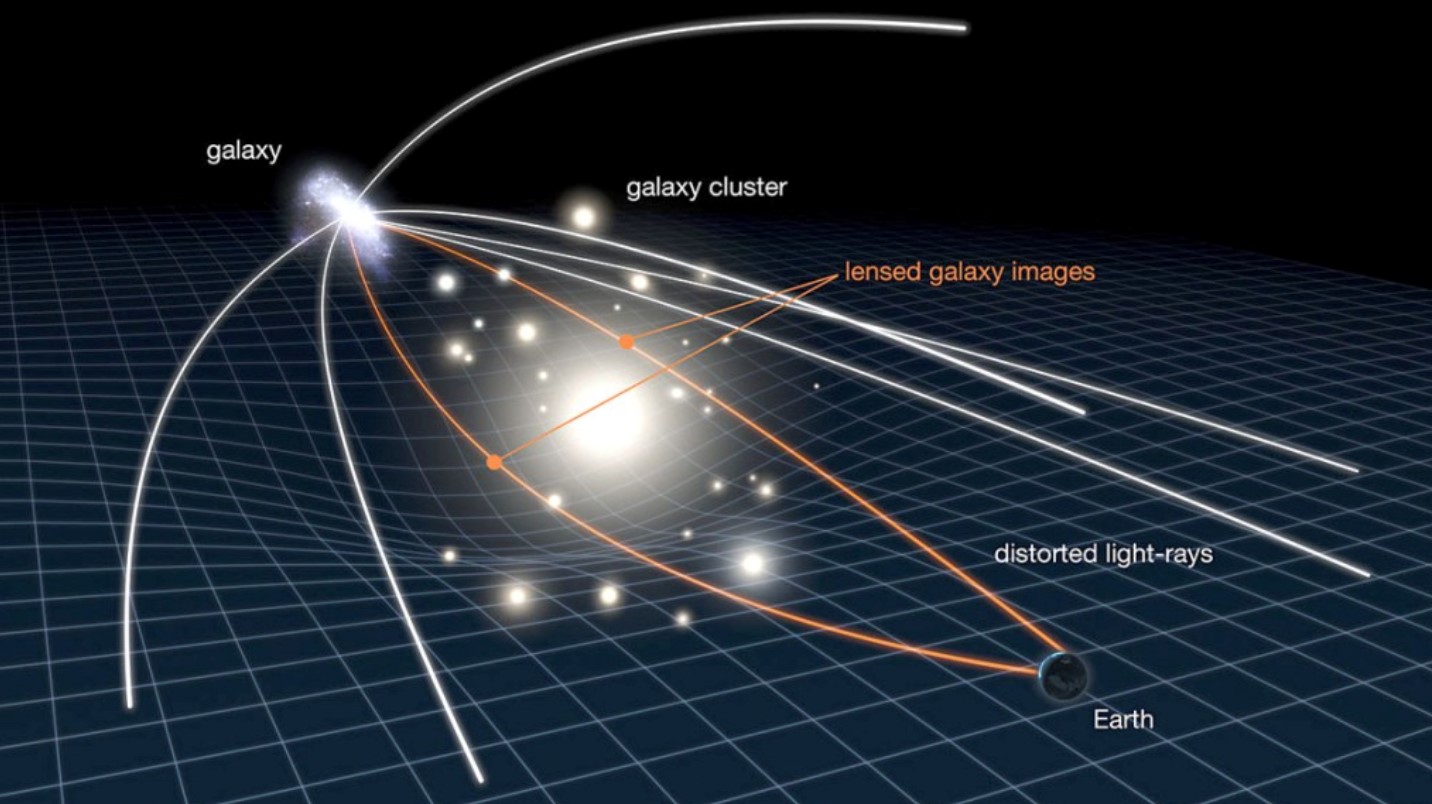
Figure 2: Trajectory of light rays moving through curved spacetime. Image credit: NASA, ESA & L. Calçada.
Galaxy clusters are the most massive objects in the universe, with masses reaching a million times a billion times the mass of our Sun. They can produce incredible distortions and magnifications on the images of objects in the background, as shown in two extreme examples in Figure 3.

Figure 3: Left: Extreme gravitational lensing by a galaxy (The Horseshoe Einstein Ring from Hubble. Image credit: ESA/Hubble & NASA). Right: Extreme gravitational lensing by a cluster of galaxies (MACSJ0138.0-2155. Image credit: ESA/Hubble & NASA, A. Newman, M. Akhshik, K. Whitaker).
Astronomers also design specific programs to maximize the probability of finding those distant galaxies. By using powerful telescopes, astronomers can produce surveys of images of massive galaxy clusters to search for the most distant objects in the observable universe. The Reionization Lensing Clusters Survey1 (RELICS) is a joint Hubble Space Telescope (HST) and Spitzer program to discover the best and brightest very-distant galaxies. RELICS used more than 100 and 800 hours of HST and Spitzer observing time, respectively, to search for distant galaxies lensed by 41 massive galaxy clusters, which were carefully selected. The program was designed by many astronomers from leading astrophysics institutions, including Dan Coe from the Space Telescope Science Institute (leading scientist/coordinator of the Hubble observations) and Maruša Bradač from the University of California Davis (leading scientist/coordinator of the Spitzer observations). These massive galaxy clusters have the highest potential to magnify the light emitted by very distant galaxies. RELICS was primarily designed and optimized to search for brightly lensed very-distant galaxies in the epoch of reionization, when galaxies started to form and ionize the neutral hydrogen in the intergalactic medium (neutral hydrogen gas that resided among galaxies).
Typical magnifications for lensed galaxies are factors of a few but can also be as high as tens or hundreds, stretching galaxies into giant arcs. Even though the RELICS program was designed to search for the most distant galaxies, anything on the background of a galaxy cluster will be magnified. Individual stars can attain even higher magnifications given fortuitous alignment between the background star, the lensing cluster, and us.
Using the images of clusters of galaxies, astronomers can model these objects in terms of their mass distributions to predict how light traveling through them will be distorted to produce magnified images of background objects. Like a guitar pedalboard that processes the clean signal of an electric guitar to produce distorted sounds, a galaxy cluster will deflect the light rays traveling through it to produce distorted images. If musicians fully understand how a pedalboard works, they can do the exercise of reversing the process to infer what the clean signal input was that produced the distorted sound output. Similarly, astronomers can determine how large was the region in the background of a galaxy cluster that produced the distorted lensed image. Recently, using this approach, several individual stars whose detected light were emitted between 7 and 9 billion years ago have been discovered, magnified by factors of thousands, temporarily boosted by perfect cosmic alignments.
Their images were detected and the sizes of the regions in the background that produced those images were computed. The sizes of those regions in the background would fit at most a few stars.
In a more recent work published in Nature2, Brian Welch (from the Space Telescope Science Institute at the time of the publication, now at University of Maryland/NASA Goddard Space Flight Center), Dan Coe, and collaborators (among them Berklee’s professor Felipe Andrade-Santos), reported Hubble observations of an even more distant star whose light was emitted 12.9 billion years ago, just 900 million years after the Big Bang, tracing back to a moment in the history of our universe when the chemical composition was very different from what it is today, given that over billions of years stars have enriched the interstellar and intergalactic environments with newly forged chemical elements. This star (bright object pointed by the arrow in Figure 4), WHL0137-LS, nicknamed Earendel (old English for morning star), is magnified by a factor of thousands by the foreground galaxy cluster WHL0137–08.

Figure 4: Earendel (pointed by the arrow) as observed by the Hubble Space Telescope. Photo courtesy of NASA, ESA, Brian Welch, Dan Coe; Image processing by NASA, ESA, Alyssa Pagan (STScI).

Figure 5: Earendel as observed by the James Webb Space Telescope. Image credit: NASA, ESA, CSA, STScI, Cosmic Spring JWST; Welch et al., 2022 ApJ, 940,1.
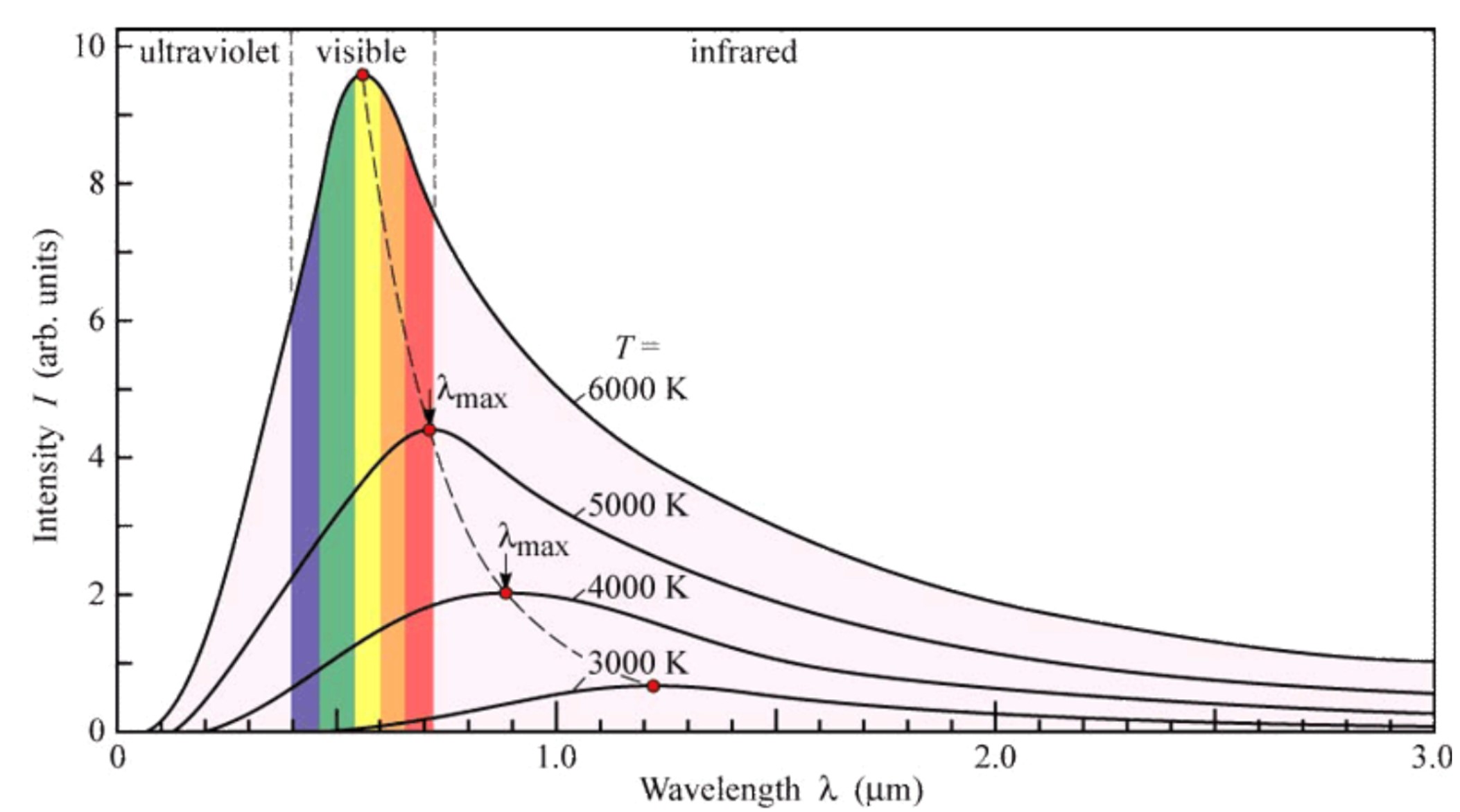
Figure 6: Black body radiation curves. Image credit: Light-Emitting Diodes (Cambridge University Press) by E. F. Schubert.
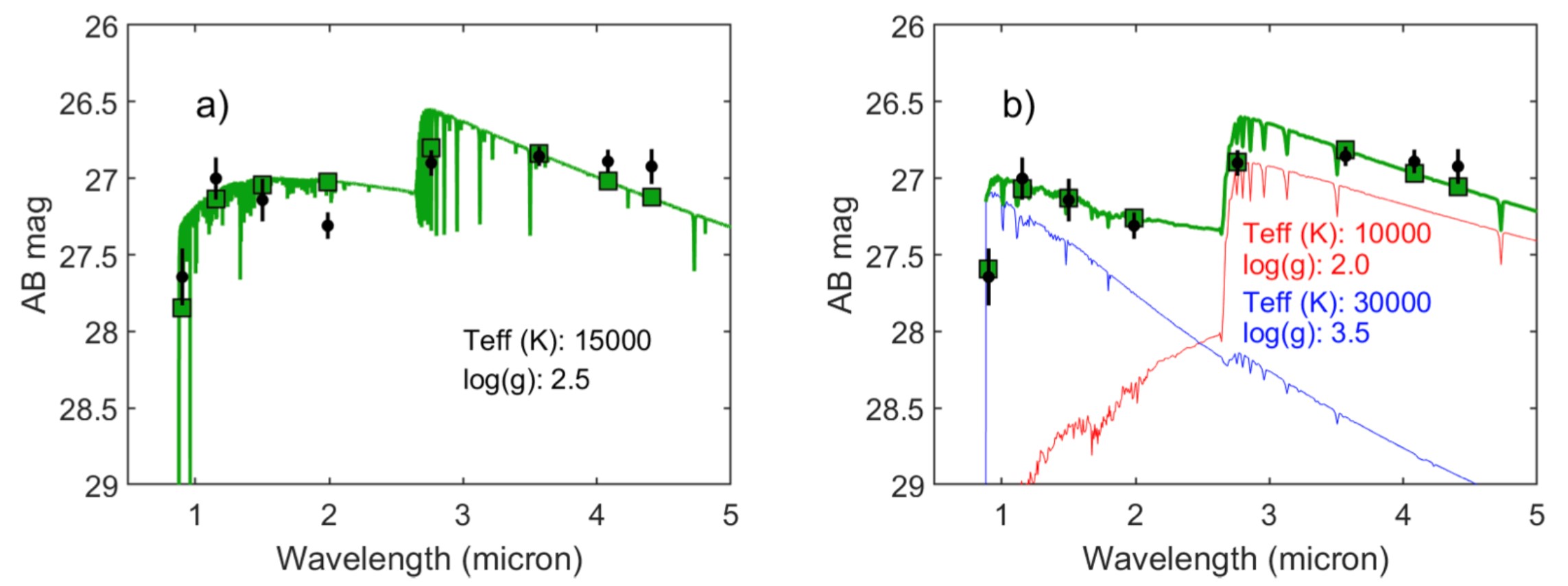
Figure 7: Earendel’s spectrum as observed by the JWST. Left: a single model is used. Right: a double model is used. Image credit: Welch et al., 2022 ApJ, 940,1.
2 https://www.nature.com/articles/s41586-022-04449-y
3 https://iopscience.iop.org/article/10.3847/2041-8213/ac9d39